Performance Testing of Ground-Fault Protective Devices
One of the most important aspects of a safe electrical power system is the proper performance of ground-fault protective equipment. Mandated by the National Electrical Code (NEC), Art. 230.95(C) states, “The ground-fault protection system shall be performance tested when first installed on-site. The test shall be conducted in accordance with instructions that shall be provided with the equipment. A written record of this test shall be made and shall be available to the authority having jurisdiction.”
The ground-fault protection system is a relatively simple system; however, if it's designed and installed incorrectly, it will not operate properly. You must conduct the performance test after the system is installed but before it is energized.
Common issues
There are many typical problems found during the testing of ground-fault systems that may prevent proper ground-fault operation. For instance, on 3-phase, 4-wire systems, the neutral conductor should not have additional grounding connections made downstream from the main bonding jumper, which must be located in the service entrance section. This condition may cause loss of sensitivity in sensing ground-fault current. Many installations are found with neutrals grounded downstream of the bonding jumper and sensor.
The neutral sensor in a residual sensor arrangement or with integral ground-fault trip on a circuit breaker may be installed with incorrect polarity with respect to the associated phase sensors. This will cause false tripping by reading balanced load current as imbalanced and interpreting this as a fault condition. Current transformer (CT) polarity issues are commonly found during acceptance testing of ground-fault systems.
The neutral conductor in a load circuit must pass through a zero-sequence sensor in the same direction as the phase conductors. Unbalanced signals cause false tripping. When a circuit is monitored by a zero-sequence sensor, none of the conductors can be omitted from passing through the sensor. Unbalanced signals cause false tripping. An equipment bonding or grounding conductor must not be passed through the window of a ground-fault sensor. This can cause unwanted cancelation of current signals and will prevent ground-fault tripping when it is needed.
Neutral grounds downstream of the sensor
Figure 1 (click here to see Fig. 1) shows what can occur during a ground fault if the neutral is grounded downstream of the ground-fault CT. The current (IF) leaves the source and flows through the ground-fault CT to the fault; however, only part of the current (IF - IFN) bypasses the ground-fault CT back to the source. Part of the current (IFN) will return on the neutral bus where it is grounded and flow through the CT. The amount of current in each of these return paths will determine how the system works. Correct operation occurs when total (IF) returns on the neutral through the CT. The system has now been desensitized. If the misplaced ground on the neutral has an impedance that's low enough, then most of the ground-fault current (IF) will flow through the CT, resulting in only a minimum unbalance — and the relay may not operate at all during a ground fault.
Figure 2 (click here to see Fig. 2) shows another common scenario when there are misplaced grounds on the neutral. Notice there is not a fault on the system in this case. However, because of the misplaced ground, part of the neutral current (ILG) is flowing through ground and bypassing the CT. If this current becomes high enough, nuisance tripping may occur. As the return current in the neutral (IL-ILG) becomes smaller, the unbalance becomes greater until a trip condition exists.
Testing neutral insulation resistance
Due to the problems created by grounded neutrals downstream of the sensing device, testing the insulation resistance is imperative. The first step is to temporarily remove the neutral disconnect link. Next, measure the resistance between the neutral and ground (click here to see Fig. 3). The value should be a minimum of 1 megohm. If the neutral is found to be less than 1 megohm, correct the problem prior to placing the equipment into service.
It is common practice to use an ohmmeter to take this reading. Use caution if an insulation resistance test set is used. Applying too high of a voltage to the neutral could damage equipment tied into the circuits downstream. Replace the neutral disconnect link after testing.
Primary injection testing
Primary injection testing consists of injecting primary current into the equipment phase and neutral conductors to duplicate the flow of ground-fault current under various conditions. This test will prove that the CTs are mounted and wired correctly — and that the switch or breaker will not nuisance trip under normal operating conditions.
Two of the most common types of ground-fault systems are the zero-sequence sensing method and the residual sensing method.
Figure 4 (click here to see Fig. 4) shows a typical zero-sequence sensor arrangement. The first step is to connect the high-current supply to one phase and to the neutral (A1 and N1). Connect a jumper between A2 and N2. Inject 1.2 to 1.5× the pickup value set on the relay. The relay should not pick up. This confirms the neutral and phase conductors go through the sensor in the same direction. Remove the jumper between A2 and N2.
Next, connect a jumper between A2 and G1. Inject 80% of the pickup value, and raise the current until the relay picks up. Record this value. This test confirms continuity of the ground path from the ground bus to the neutral as well as proper operation of the relay.
The timing test requires testing the time delay of the relay. This is accomplished by testing the system at 2.0× of the pickup value. Inject current at this value, and record the trip time. Compare the trip time against the manufacturer's curve. Remove the jumper between A2 and G1.
For a zero-sequence sensing system, these are the only high-current tests required. It's not necessary to repeat the tests for each phase if a visual inspection confirms that all phases go through the sensor window in the same direction.
Figure 5 (click here to see Fig. 5) shows a typical main breaker with ground-fault relay and residual sensor arrangement. Connect the high-current supply to A1 and N1. Place a jumper between points A2 and N2. Inject 1.2 to 1.5× the pickup value set on the relay. The relay should not pick up. This confirms the polarity of the sensor connections. If pickup would have occurred at 50% of expected value, this is an indication that the neutral sensor polarity is reversed. Remove the jumper between A2 and N2.
Next, connect a jumper between A2 and G1. Inject 80% or pickup value and raise the current until the relay picks up. Record this value. This test confirms continuity of the ground path from the ground bus to the neutral as well as proper operation of the relay.
The timing test requires testing the time delay of the relay. This is accomplished by testing the system at 2.0× of the pickup value. Inject current at this value, and record the trip time. Compare the trip time against the manufacturer's curve. Remove the jumper between A2 and G1. Repeat these tests using the connections shown in the Table (click here to see Table).
Testing reduced control voltage tripping capabilities
Many ground-fault systems require control voltage to operate, and many systems have control power transformers that supply the control voltage. This is advantageous, because the ground-fault system is a stand-alone system that does not require an additional source to operate. The disadvantage is the control power can be reduced during a ground fault; however, the equipment must be able to operate on the reduced voltage to clear the fault.
For example, the control power for a particular system is derived from a 480V/120V control power transformer (click here to see Fig. 6). The transformer is energized from the incoming voltage of the gear the ground-fault system is protecting. The transformer is connected through a set of fuses to “A” and “B” phases — 480V on the primary of the transformer and 120V on the secondary.
If a ground fault occurs on “A” phase, then “A” phase is at ground potential during the fault. The control power transformer now has “B” phase — and ground on the primary of the transformer or 277V. The secondary voltage is reduced as well. The secondary or control voltage of the system is now at 277V ÷ 4 = 69V. The system must be able to operate at 69V, approximately 58% of the nominal control voltage.
It's standard practice to test the system at 55% of the rated control voltage for AC systems. The ground-fault relay and circuit breaker or switch must operate at this reduced voltage; therefore, it must be tested at the reduced control voltage. If the system does not operate at the reduced control voltage, the system should be repaired prior to placing into service.
In overview
Performance testing of a ground-fault system must include primary injection, neutral insulation resistance, and reduced control voltage testing to ensure the system is installed and operates properly. These tests are not included in the push to test (see Ground-Fault System Testing Using Simulated Fault Current (Push to Test) or simulated fault current method of testing a ground-fault system.
Ground-fault protection systems are important because they're deemed necessary to provide a safe and efficient electrical power system. However, the system must operate properly for this end result to occur. The only way to know the system is designed and installed properly is to follow Art. 230.95 requirements during installation and properly performance test the system prior to energizing it.
Editor's Note: The original content of this article was presented at the PowerTest Conference held February 2010 in Long Beach, Calif. (www.powertest.org).
Beisert is production manager of the engineering service division at Shermco Industries, Irving, Texas. He can be reached at [email protected].
Sidebar: Ground-Fault System Testing Using Simulated Fault Current (Push to Test)
The simulated fault current method uses a simulated fault current generated by a coil around a window-type sensor or by means of a separate test winding in the sensor. When the monitor panel sends a small current through the test winding (push to test), a secondary current is produced in the relay, which the relay responds to as if it were caused by a primary current.
Testing with simulated fault current provides a means of demonstrating the operation of the sensor, relay, shunt trip, and the adequacy of the control power supply. However, it does not confirm that neutral ground points are located correctly with respect to sensors, sensor polarities are correct, and all conductors that pass through a sensor window run in the same direction. Therefore, the simulated fault current method is not adequate to test the entire system and cannot prove the system is installed correctly.
Voice your opinion!
To join the conversation, and become an exclusive member of EC&M, create an account today!
Sponsored Recommendations
Latest from Maintenance, Repair & Operations
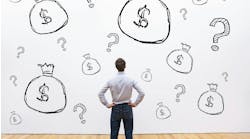